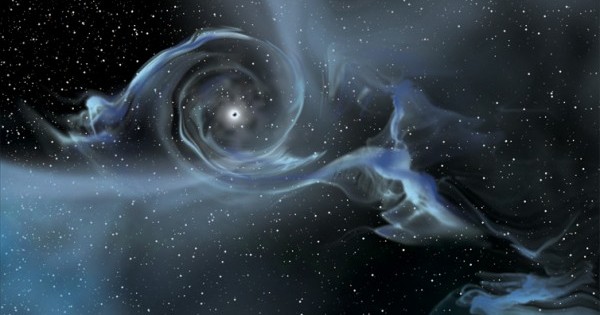
Black holes are heavy things. We can’t see them, but we can infer their existence from their gravitational effects on their neighborhoods. Because light can’t escape them, they’re dark, very dark.
A black hole has a border, a fence, a boundary, a line on a map, called the event horizon. If you were to cross the event horizon you would be gone, never to be seen again. Goodbye. This is the case for everything that drops into a black hole. Black holes do accrete, but they don’t suck. They’re not vacuum cleaners.
The event horizon is the point at which the escape velocity is greater than the speed of light. Escape velocity is the speed required for an object such as a rocket to escape the gravity of wherever it’s departing from. Nothing travels faster than light, but light does not travel fast enough to escape a black hole.
A black hole typically begins with a neutron star—the remains, the core, the corpse of a massive supernova—a massive star that has exploded. Keyword: massive. Only stars at least 10 times as big as our Sun are massive enough to go supernova and leave a neutron star.
A tremendous force of gravity bears down on the core of stars, but they don’t collapse because something is pushing back. That something is nuclear fusion. It starts with hydrogen, the element that makes up 75 percent of the universe. Four hydrogen atoms, each with one proton, fuse to make one helium atom with two protons and two neutrons. The excess energy escapes as heat and light. This goes on for millions of years. But eventually the hydrogen at the star’s core is used up, and then helium atoms at the core begin fusing to make even heavier atoms—carbon and oxygen. After the helium is used up, the carbon and oxygen begin fusing, and so it goes. Each fusion event releases heat and light because the new, heavier element’s atom is a bit lighter than the sum of its predecessor atoms. Fusion continues until the core is iron. Only the most massive stars have enough gravity to create enough heat and pressure to force fusion all the way to iron.
Iron can’t liberate energy because fusing to iron absorbs energy. Iron can’t release heat and light to oppose the force of gravity. All the iron core can do is get heavier.
So gravity wins, and the star collapses and, in a kind of trampoline effect, explodes. It goes supernova. The outer layers disperse to space. The core remains, spinning, spinning, spinning. This is a neutron star. It’s small—perhaps six miles wide—but exerts gravity so extreme that a teaspoonful weighs more than Mount Everest. Its magnetic field is a trillion times as strong as that of Earth. As it spins it emits radiation in the form of pulsars (think of a revolving lighthouse beacon flashing by at regular intervals). In 1967, astrophysicist Jocelyn Bell, then a graduate student, discovered pulsars and thus neutron stars, which had been predicted in theory but never found.
Neutron stars are heavy, but within them something potent (not fusion) pushes back against the force of gravity. So extreme is a neutron star’s gravity that each proton with its positive charge latches onto each electron with its negative charge, and what you get is neutrons—subatomic particles with no charge. Here quantum principles come into play: when particles are mashed together as close as nature permits, no two neutrons can occupy the same place at the same time; nor can they exist in the same quantum state. This creates “neutron degeneracy pressure” that pushes back against the extreme force of gravity.
But throw more stuff into it. Make it heavier. Bet on gravity. This can happen in binary star situations where the neutron star slurps matter from its companion luminary. At a certain point, gravity wins again, this time victorious over neutron degeneracy pressure. The star collapses into itself, perpetually and forever. Now you have a black hole.
A black hole puts a dip in the fabric of space-time. Einstein’s General Theory of Relativity holds that what we perceive as gravity is the warping of space and time produced by energy and matter. Astronomy books have you picture a flat rubber sheet with a heavy object pressing down into the center (the black hole) with the rest of the sheet sloping down toward the hole, causing objects spinning around the slope to spin faster. The rubber-sheet image works, except a rubber sheet is two-dimensional and space-time is four-dimensional. I prefer the image of a banked-track roller derby with roller-girl teams like the Hellcats or the Hired Guns whipping around at near the speed of light. (I refer, of course, to the Lonestar Rollergirls of Austin, Texas.)
But I digress. Gravity affects time, slowing it down. This property of time was predicted by the General Theory of Relativity, and is no longer controversial. It has been tested by flying accurate clocks high above Earth where gravity is weaker. As expected, up there, time speeds up. Conversely, at the edge of a black hole, gravity is so great that time stops. Bam!
Here you may be tempted to ponder strange strings and wondrous wormholes and ultra-weird alternate universes where teams like the Throttle Rockets of Seattle’s Rat City Rollergirls don’t even exist. You may be tempted but I am not. Goodbye.